FINDING PLANET NINE: A Resonance Modelling Approach
A Science Poster for Master Project: Computational Astrophysics
Heidi Kneale, Swinburne University
100247548@student.swin.edu.au
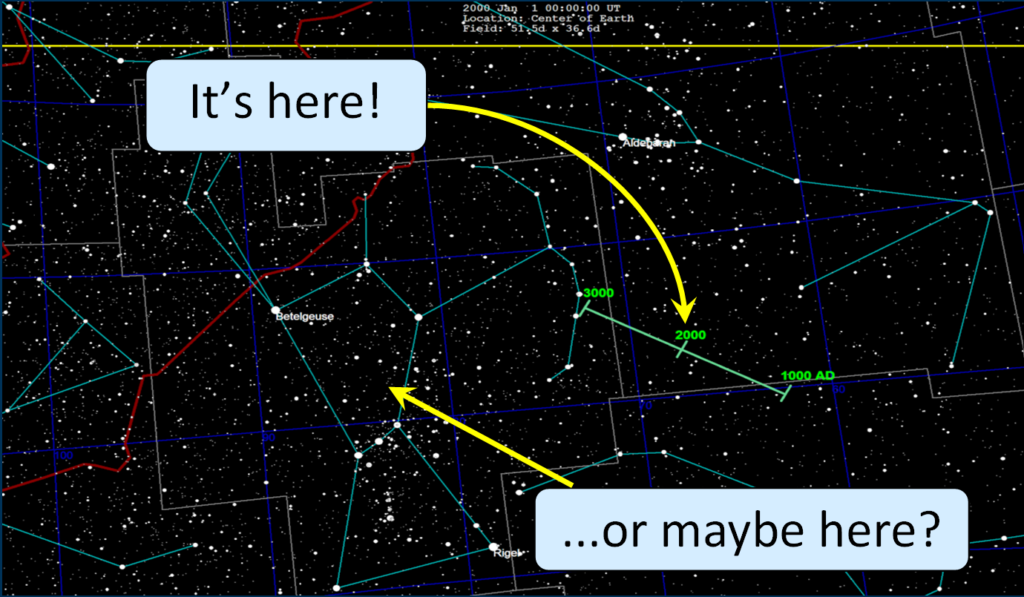
Image credit: Tom Ruen (commons.wikimedia.org —CC BY-SA 4.0, altered)
Overview
The outer-outer Solar System, with no other planets beyond Neptune, should be quiet, stable and flat. But with the discovery of objects out beyond the Kuiper Cliff demonstrating wild eccentricities and steep inclinations, something needs to explain how they got that way.
In 2016 Konstantin Batygin & Michael E. Brown presented a hypothesis that the Solar System could have a ninth major planet well beyond the orbit of Neptune (Batygin and Brown 2016). They call it Planet Nine. They derived its potential orbit and mass based on apparent coincidences in certain orbital parameters of a half-dozen extreme trans-Neptunian Objects (eTNOs). While they’ve been able to constrain the orbit of Planet Nine somewhat, to this date it has not yet been visually confirmed (Batygin et al. 2019). But they believe it is out there. If it is, it will have certain gravitational effects on outer-outer Solar System objects. Since 2014 (Trujillo & Sheppard 2014), the presence of more and more eTNOs that appear affected by a long-term gravitational perturber have been confirmed. If Planet Nine is in a certain orbit, many eTNOs will behave in certain ways. Define these effects, and you’ve got an arrow pointing to Planet Nine’s orbit.
One way to work out Planet Nine’s orbit is to study its effect on outer-outer Solar System objects—essentially, defining a population we call “Niners”.
Through computer modelling we’ve created various scenarios to better understand the potential effect of Planet Nine’s gravity on these objects (Kneale 2020). Once those effects are known and parameters established for the different models, outer-outer Solar System objects can be compared to these models to help refine Planet Nine’s effects on Niner objects in a real-life scenario. For example, the mean-motion resonance effects of Planet Nine at an SMA of 400AU will look different than at an SMA of 800AU.
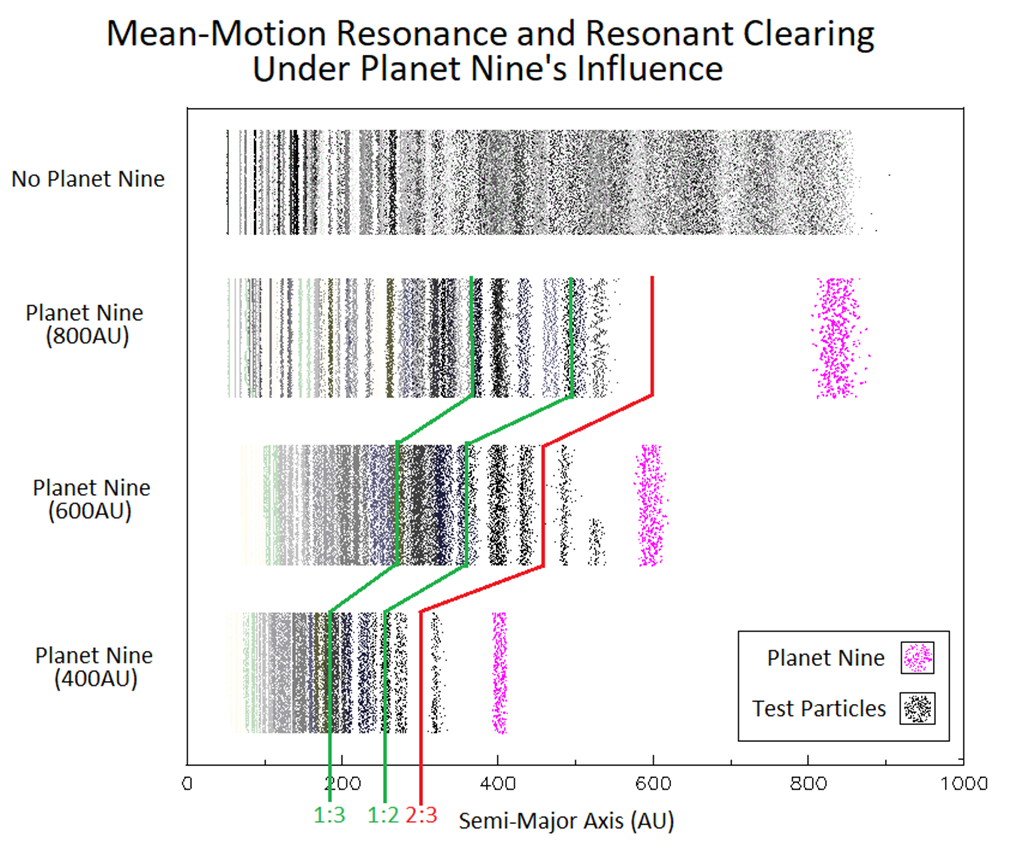
Without a gravitational perturber, the outer-outer Solar System doesn’t clear out or sort into resonances. With Planet Nine (purple), resonances at 1:3 and 1:2 populate nicely while the 2:3 clears out. For the model at 400AU, resonant clustering started at about 180AU, with a clear-out happening within 100AU+ of Planet Nine’s SMA. Planet Nine appears to clear its neighbourhood. The location of these populated and cleared resonances can provide further clues as to Planet Nine’s actual semi-major axis.
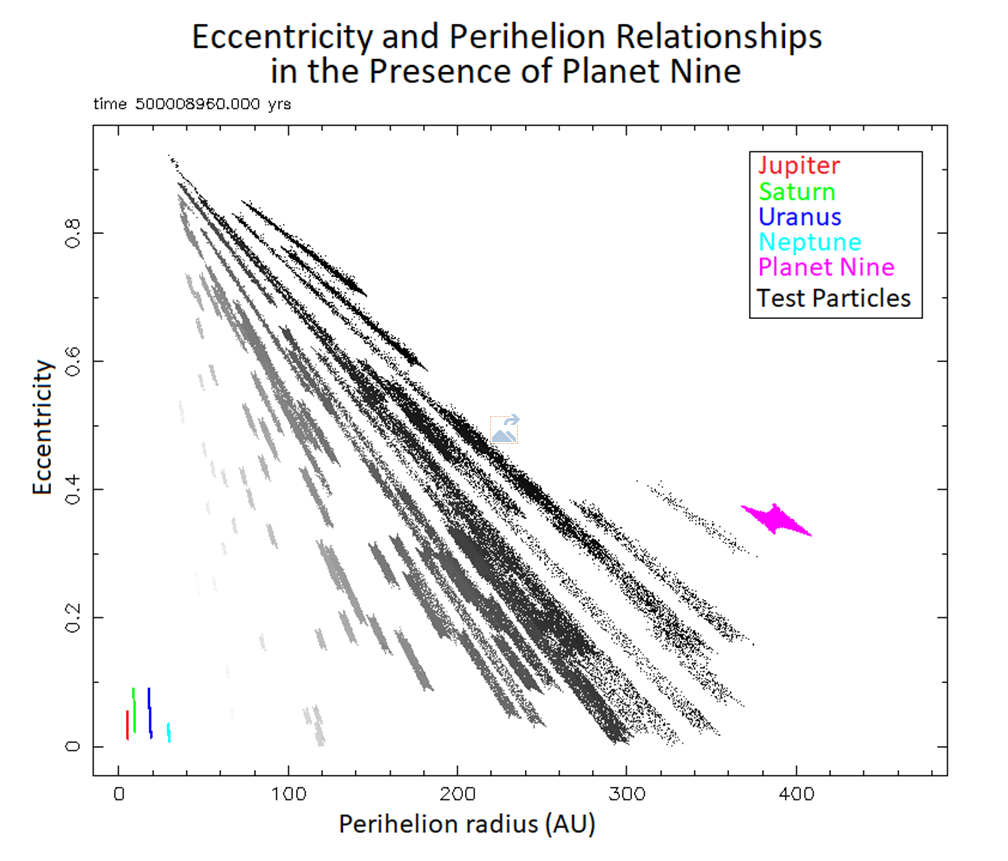
The relationship between eccentricity and perihelion radius marks Planet Nine’s effect on the Niner population. When eccentricity rises, the perihelion drops. If an object has a high eccentricity, chances are the perihelion is close to its minimum distance. If that object’s perihelion is outside of Neptune’s influence, it’s probably a Niner.
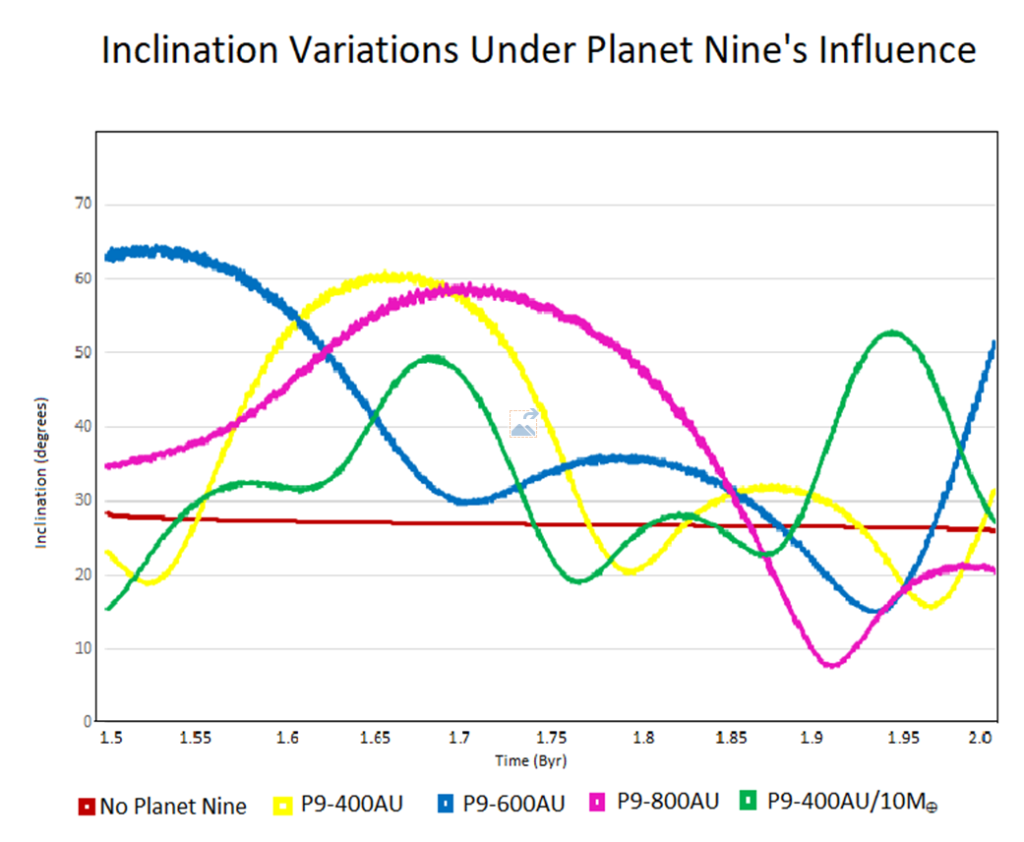
With no perturber in the outer-outer Solar System, objects remain in the same inclination, fossilised in their orbits (see red line). But introduce Planet Nine into the model, and it has the capability of lifting inclinations quite significantly. The wave formations show that these objects are not flung from the Solar System, but are retained in a resonance. An object in a bound orbit with a high inclination is probably a Niner.
You might have a Niner if…
- SMA mean-motion resonances are greater than 180AU
- Perihelion is detached from the influence of Neptune
- Eccentricity and inclination are connected in an oscillating resonance pattern
- Orbital precession is present due to orbital resonant oscillation
- A high-low relationship exists between eccentricity and perihelion (if one is low, the other is high)
- Clustered arguments of perihelion will match with other objects in a similar plane
Planet Nine simulations were generated on the Solar System Dynamics simulator, based on the SWIFT code (Levison & Duncan 1994) on the OzSTAR Swinburne supercomputer (Kneale 2020).
Bibliography
- Batygin, K., Adams, F. C., Brown, M. E., & Becker, J. C. 2019, Physics Reports, 805
- Batygin, K., & Brown, M. E. 2016a, AJ, 151
- Kneale, H 2020, AST80011 project report, submitted to Swinburne
- Levison, H. F., & Duncan, M. J. 1994, Icarus, 108, 18
- Trujillo, C. A., & Sheppard, S. S. 2014, Nature, 507
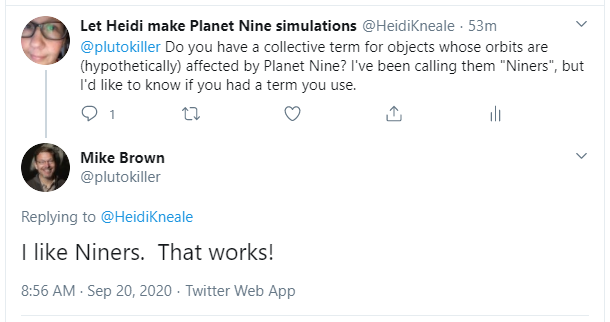